Our Innovation
OPTIC's integration of Quantum-Dots combined with cell adhesion molecules and microelectrode arrays achieves the effect of an accurate replication of vision while additionally remaining flexible and minimizing possible damage. OPTIC vastly improves multiple aspects of a retinal prosthesis, including stability, future growth, and implementation, allowing for a safe and accurate retinal prosthesis.
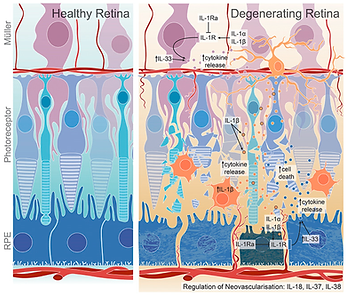
The diagram above depicts both a healthy retina (left) along with a degenerating retina (right) affected by inflammation in retinal neurodegeneration and degenerative diseases.



CAD and Hand-Drawn
Representations
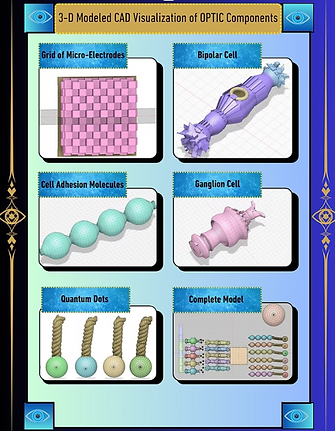

Quantum Dots
OPTIC utilizes Quantum-Dot Based Photoreceptors that mimic the function of light-sensitive natural photoreceptors. In addition, Quantum-Dots are able to successfully transmit signals through to the electrode arrays at similar frequencies to naturally occurring signals from photoreceptors. This allows Quantum-Dots to replace the missing or damaged photoreceptors in individuals.
Cell Adhesion Molecules
The Quantum dots are connected to remaining retinal cells (if any) using specialized proteins; otherwise, they will be integrated directly into the adjacent retinal tissue using the same type of protein. To ensure precise alignment with the cells and tissue, the optimal materials to employ would be Cell Adhesion Molecules (CAMs) N-cadherin and E-cadherin. These particular proteins meticulously facilitate cells in adhering to each other and/or their environment.
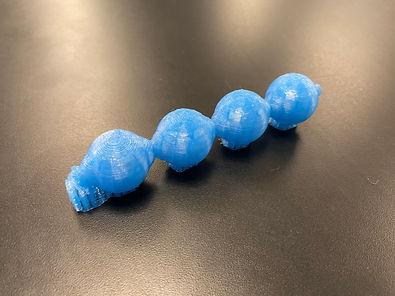
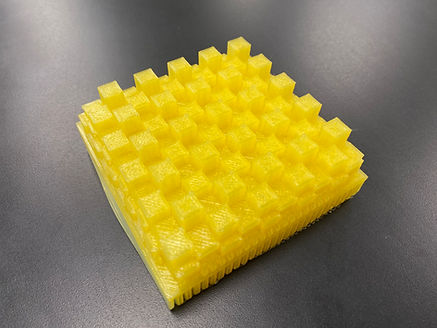
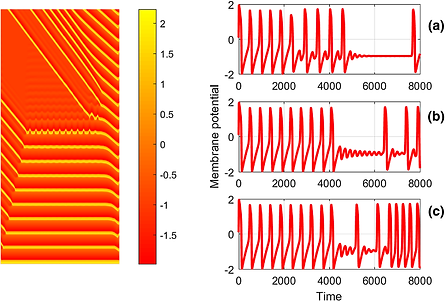
Grid of Microelectrodes &
Spatiotemporal Pattern Dynamics of Membrane Potential
Due to the retina's delicate and curved surface, OPTIC integrates Sub-Micron Flexible Electrode Arrays that are similar to usual electrode arrays used in past retinal prosthetics, but utilizes flexibility to minimize damage or irritation. Through these electrode arrays, OPTIC is able to stimulate retinal neurons and encode visual information from the Quantum-Dots. The visual information is encoded as spatiotemporal patterns of electrical impulses. These electrical impulses mimic the natural signaling patterns of photoreceptors- a format that retinal ganglion cells can interpret and transmit to the brain via the optic nerve for visual perception.
The first image demonstrates the CAD-made structure of the microelectrode array, this allows for precise stimulation across the curved retinal surface. The second image illustrates the Spatiotemporal Pattern Dynamics of Membrane Potential. This depicts how the electrical signals vary across space and time. This also reflects how OPTIC encodes dynamic visual stimuli. Rather than just light detection and conversion, it also adapts to the changing signals over time. These patterns allow the encoded data to be interpreted accurately by bipolar and ganglion cells. The result? High-fidelity visual restoration.
Bipolar Cells
Bipolar cells receive this input through synapse-like interfaces with the electrode array. The encoded electrical impulses mimic the graded potentials naturally produced by photoreceptors, meaning that the electrodes don’t just fire all-or-nothing action potentials — they reproduce variations in signal strength and timing. This enables the bipolar cells to detect subtle differences in light intensity, contrast, and motion, just like in a healthy retina.
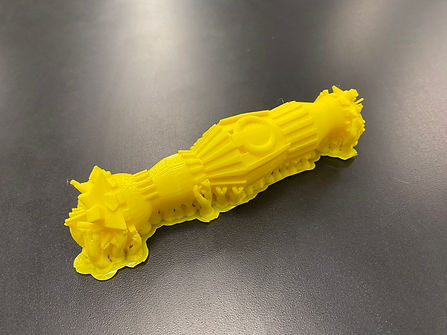
Bipolar-Ganglion Cell Transmission
Bipolar cells then transmits the graded electrical signals, who's strength varies based on the intensity of light, through synapses in the inner plexiform layer. The usage of this precise communication ensures that the ganglion cells receive accurate visual information to send to the bipolar cells.


Ganglion Cells
Ganglion cells are the final output neurons. Once they receive and process the signals from the bipolar cells and convert them into action potentials, the electrical impulses travel through the optic nerve to the brain’s visual cortex. This allows for visual perception to occur.
Ganglion Cells to Optic Nerve

The ganglion cells generate action potentials that travel down their axons once they receive the visual information from bipolar cells.
The process of signal transmission is influenced by retinal signal propagation:
1. Embryonic Waves:
Early electrical activity in the retina that establish connections between retinal cells.
2. Cholinergic Waves:
Acetylcholine created waves that strengthen the retina’s network and synaptic connections.
3. Glutamatergic Waves:
Waves created by glutamate that refine the processing of visual signals.
Optic Nerve
The optic nerve sends visual information from the retina to the brain. Furthermore, this nerve transmits electrical signals to the visual cortex, in which the brain processes and interprets the signals. This allows us to perceive vision efficiently.
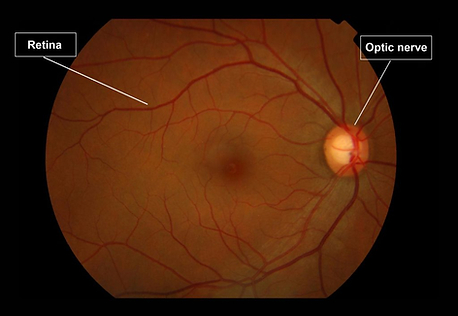
Optic Nerve Signal Conduction
The optic nerve signal conduction incorporated the transmission of action potentials from the ganglion cells to the brain. The axons of ganglion cells are covered with myelin which is a fatty substance which helps by speeding up electrical signal transmission. The signal then goes between Nodes of Ranvier which are essentially small gaps in the myelin sheath. These allow for faster conduction through saltatory conduction. The segments of the axon between these nodes are called internodes. Within this area, myelin insulation ensures efficient transmission of the signal.
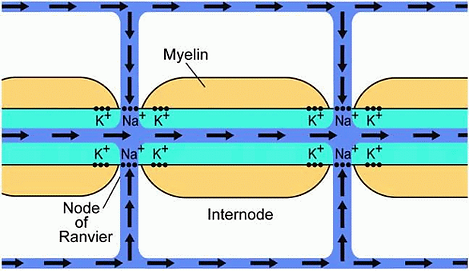